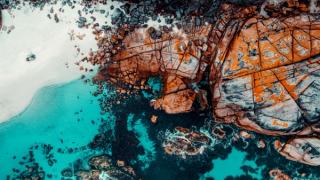
Climate Transition Plans: Decarbonisation is only one part of the puzzle
by James Balik-Meacher
View post
Whether we are driving an electric car, zipping around on an e-scooter, or just using our cell phones, most of us use devices with rechargeable batteries every day.
These batteries contain critical elements such as cobalt, nickel, and manganese, all of which are difficult to access. As the demand for this technology grows, the need for these elements is also on the rise and is expected to triple by 2030.
As a result, great interest has turned towards the deep-sea ocean seabed, where such elements can be abundant in polymetallic nodules found on or under the seafloor (Figure 1). Due to the great depths involved (up to 6000 m), the process of nodule collection, known as Deep Sea Mining or DSM, occurs in multiple stages on the seafloor, over the water column, and on the surface. Each step generates underwater noise that can have a significant impact on marine life.
The three main mineral resources (Hein et al. 2013) in Deep Sea Mining are:
Deep-sea habitats include canyons, plains, mountains, trenches, hydrothermal vents, methane seeps, wood falls, whale falls, brine pools, and the deep pelagic zone. These habitats are home to many species, from microscopic bacteria to deep-diving large marine mammals (Miller et al., 2018).
To access these resource-rich polymetallic nodules, nodule mining is conducted via a seafloor collector (see Figure 2) that moves along the seabed.
Nodules pass from the collector to a vertical transport system (VTS), which lifts the nodules as a slurry to a production vessel (PV) equipped with a Dynamic Positioning System (DPS) and are then connected to the subsea equipment.
Once onboard the PV, nodules are dewatered, transferred, and stored.
Water from the dewatering process is recycled and discharged via a pipe on the VTS. Nodules will be transferred from the PV to an Ore Transport Vessel (OTV). The OTV will export the mixture of nodule ore to a processing plant. All metals found in the recovered nodules may be refined and sold.
Other traffic related to the Deep Sea Mining process includes supply vessels arriving at the PV (re-supplying the vessel with personnel, food, fuel, and other supplies) and crew transport vessels for personnel movements.
Sound emissions during Deep Sea Mining can originate from the surface or below and includes all acoustic exploration methods (such as sonar and seismic airguns), production vessel/platform propulsion, and DPS, as well as sound from machinery & pumps, onboard treatment of ore, offtake vessels, supply vessels, and monitoring vessels.
Acoustic exploration, extraction, machinery, submersibles, ROVs, and AUVs are known sound sources related to Deep Sea Mining activities on or near the seafloor. In addition, buffer stations and subsea lift pumps, which comprise the sub-surface/near-seafloor components of the riser system (Figure 2), are additional sources of underwater sound.
Sound emissions from nodule mining activities will result in vibrations in the seabed from the dredging process and the collector device itself (Theobald et al. 2019). Peer-reviewed sources identify sound emissions from polymetallic sulfide mining, with one estimation indicating levels of 185 dB (re 1 μPa @ 1 m) with a temporal scale of weeks for drilling and 195 dB (re 1 μPa @ 1 m) with a temporal scale of months up to years for scraping along rock or rock sub-crops (Lin et al. 2019). The latter is similar to the source levels measured from large tankers with an average level of 186 dB and a maximum of 192 dB (Roberts Bank Terminal 2 Technical Report, 2014). Or well equivalent to the sound emissions of thunder (~125 dB re 20µPa @ 1 m).
Marine animals, including those in the deep sea, are confronted with threats, such as Deep Sea Mining, fisheries bycatch, habitat degradation, chemical pollution, marine litter, vessel strikes, and climate breakdown, which rarely occur in isolation.
With Deep Sea Mining, marine biota face several stress-inducing factors, including underwater sound, habitat destruction, plumes, chemical pollution, and light pollution. Exposure to underwater noise can lead to behavioural and physiological impacts on marine mammals. Behavioural responses to noise included changes in marine mammal vocalization, rest, diving and breathing patterns, and avoidance of noise sources. Masking (or overlapping of acoustic frequencies used by marine mammals by anthropogenic noise sources) of biologically important sounds may interfere directly with communication and social interactions. Secondary behavioural effects such as inhibited reproduction cycles and other changes in behaviour may also occur.
Physiological effects of underwater noise are primarily associated with the auditory or hearing system. Exposure to a high level of noise for a specific duration can cause a reduction in the animal's hearing sensitivity. Suppose the noise exposure is below a critical sound energy level (determined by grouping marine mammals into functional hearing groups based on frequency range). In that case, the hearing loss is generally only temporary, an effect known as a Temporary Threshold Shift (TTS). Hearing loss can be permanent if exposure to underwater noise levels exceeds a critical sound energy level. This effect is known as a Permanent Threshold Shift (PTS).
Other environmental effects, such as the disturbance of seafloor sediment resulting in the spread of toxic heavy metals, can occur. In addition, light from mining could affect marine life and impact habitats on the ocean floor, around hydrothermal vents, and on mountains and sea ridges.
These effects may combine so that the impact on marine life is greater than the sum of the individual ones. While an animal may be able to cope with one stress factor from Deep Sea Mining, it could quickly be overwhelmed by many at once.
Mutual effects have already been suggested for dredging-related stressors, where the effects of contaminated sediment were significantly greater than studies on clean deposits alone or the generation of underwater radiated sound (Wenger et al. 2017). However, additional research is required on the long-term effect of habitat loss for these creatures and how that loss might affect ecosystems and people.
Deep-sea mining is a potential source of critical and other minerals that may enable innovative technologies such as extended-range electric cars, lighter rechargeable batteries, and more weather-resistant wind turbines. However, as this technology matures, policymakers and stakeholders should consider its benefits and environmental costs. Assessing cumulative underwater noise levels and corresponding impacts (whether TTS or PTS in nature) on marine mammals is essential in understanding the effects of deep-sea mining on marine life.
When considering a project involving marine life, we recommend that a sound transmission loss modelling assessment is done, including the following:
For more information about these services, please get in touch.
References:
Hein, J. R., Mizell, K., Koschinsky, A., & Conrad, T. A. (2013). Deep-ocean mineral deposits as a source of critical metals for high- and green-technology applications: Comparison with land-based resources. Ore Geology Reviews, 51, 1-14.
Lin, T.-H., Chen, C., Watanabe, H.K., Kawaguchi, S., Yamamoto, H., and Akamatsu, T. (2019). Using soundscapes to assess deep-sea benthic ecosystems. Trends in Ecology & Evolution 34, 1066-1069. doi: 10.1016/j.tree.2019.09.006.
Miller, K.A., Thompson, K.F., Johnston, P.A., & Santillo, D.G. (2018). An Overview of Seabed Mining Including the Current State of Development, Environmental Impacts, and Knowledge Gaps. Frontiers in Marine Science, 4.
Roberts Bank Terminal 2 Technical Report (2014). Underwater Noise Ship Sound Signature Analysis Study. Prepared for Port Metro Vancouver. Prepared by Hemmera Envirochem Inc., SMRU Canada Ltd. and JASCO Applied Sciences (Canada) Ltd.
Theobald, P.D., Lepper, P.A., Robinson, S.P., Hayman, G., Humphrey, V.F., Wang, L.-S., Mumford, S. (2019). Underwater Noise Measurement of Dredging Vessels During Aggregate Extraction Operations. figshare. https://hdl.handle.net/2134/9597
Wenger, A.S., Harvey, E., Wilson, S., Rawson, C., Newman, S.J., Clarke, D., Saunders, B.J., Browne, N., Travers, M.J., Mcilwain, J.L. and Erftemeijer, P.L. (2017). A critical analysis of the direct effects of dredging on fish. Fish and Fisheries, 18(5), pp. 967-985.
by James Balik-Meacher
by Peter Lo
by Melissa Birch, Tarra Tamang